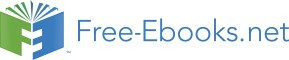

bermudagrass ( Cynodon dactylon (L.) Pers.), bahiagrass ( Paspalum notatum), atra paspalum ( Paspalum atratum Swallen), digitgrass ( Digitaria decumbens) (En), limpograss ( Hemarthria 36
Agricultural Science
altissima), and Rhodes grass ( Chloris gayana (Kunth)) (Verdramini et al., 2010). All of these grasses except atra paspalum, bahiagrass and Rhodes grass require vegetative propagation
in order to be established. As with temperate pastures, the pasture must first be properly prepared by controlling weeds, insects, removing old or dead vegetative material and
fertilizing to soil test recommendations. Burning is often used in preparing bermudagrass pastures for renovation (Stichler and Bade, 2005). Tillage operations are often performed during dry periods in the spring to further control weeds and old sward growth as well as better prepare the land for vegetative propagation with the onset of summer rains (Stichler and Bade, 2005;Verdramini et al., 2010). In both the subtropical and temperate climates
warm season annual forages may be included into crop rotations on land used primarily for cultivated crops. This is to provide additional pasture, hay or silage for livestock
operations. Species such as pearl millet ( Pennisetum glaucum (L.) R.Br.), sudangrass
(Sorghum bicolor subsp. Drummondii (Steud.) de Wet ex Davidse), and other forage sorghum and sorghum x sudangrass crosses often fill this role (Hancock, 2009).
6. Summer fallow
Summer fallow, the practice of growing a crop every other year and in most systems,
controlling weed growth during the off-year, has been used almost exclusive in semi-arid
regions for the production of wheat or other small grains. Its purpose is to accumulate
sufficient limited rainfall during the fallow year to grow a crop the following year and to break crop disease cycles. Most of the world’s drylands are in developing countries where water resources are usually limited by a lack of rainfall and potential irrigation (Ryan et al., 2008). Stewart et al. (2006) has stated that dry regions worldwide supply about 60% of
human food stuffs. Areas where this is a common practice are the Mediterranean, semiarid regions of Africa, Asia, Australia, the Pacific Northwestern United States, and the western regions of the Canadian and United States Great Plains. Many of these areas practice a
“clean” summer fallow where the land is kept weed free during the fallow year, usually by cultivation or herbicides. However, in northern Africa a weedy fallow is often employed
where palatable weeds and volunteer crop plants are allowed to grow and then be grazed
by livestock (Ryan et al., 2008). This allows for an animal agriculture to exist that provides needed calories and returns manure to the land to provide nutrients for the following crop.
In semiarid areas of the Pacific Northwestern United States summer fallow wheat
production has been practiced nearly 130 years (Schillinger et al., 2007). In recent years though, studies have been conducted in semiarid regions of the United States and Canada to adapt a continuous cropping system to replace summer fallow. Water storage efficiency in most summer fallow-wheat rotations is usually less than 25% of the rainfall received during a 14 month period using conventional tillage (McGee et al., 1997). Research from Colorado and Nebraska demonstrated that precipitation storage efficiency could be improved to 40%
to 60% through minimum or no-till which allows crop residue to remain on the soil surface and soil disturbance is held to a minimum or eliminated (Croissant et al., 2008). However, such practices added little or no increase in wheat yields and it was concluded that the
resultant water savings could only be converted to profit by employing intensive cropping systems where fallow time is decreased and summer crops such as maize, grain sorghum, or
annual forages and included in the rotation. Under no-till it was determined that it cost more to save the additional water than the value of the added grain yield. Lyon et al. (2004) Concepts in Crop Rotations
37
reported that winter wheat yields in the central Great Plains were negatively affected by eliminating the 11 to 14 month summer fallow by spring planting a transitional crop before wheat in the fall. However, a spring planted forage crop that was harvested early had a
minimum negative impact on wheat yields and that the value of the forage combined with
the following wheat yields resulted in greater income than the traditional winter wheat-
fallow rotation. Research from the Horse Heaven Hills region of Washington looked at a
continuous no-till hard red spring wheat system verses a winter wheat-fallow rotation and found the hard red spring no-till system did not match the winter wheat-fallow system of
production in yield or income. However, the continuous no-till system did offer a benefit of providing ground cover that reduced wind erosion and air pollution by dust particles
(Young et al., 2000).
Summer fallow is also practiced on fields used to produce castor bean ( Ricinus communis L.) the previous year. This is not done for accumulating moisture but to rid the field of any volunteer plants. Castor bean contains a very deadly toxin, ricin, which in very small quantities can kill humans and livestock. Summer fallow in this case allows volunteer
plants to be destroyed and the field cleaned for future feed and food crops.
7. Organic farming and crop rotations
Organic farming has embraced crop rotations as its backbone to success. Crop rotation is practiced with what appears to be, much more intensity than most conventional farming
systems with particular emphasis on sustainability. A crop rotation plan and accompanying records for a field and/or farm are required for certification as an organic farming operation (Johnson and Toensmeier, 2009). Organic farming and its use of crop rotations could be
summed up in part, as the employment of proven crop management practices prior to the
advent of pesticides and processed fertilizers. This is not to say that improvements on those rotation systems have not occurred. Most have been modified to accommodate
mechanization and most other time saving ideas. But, yields may be lower. Maeder et al., (2002) reported on a 21 year study in Europe that crop yields on organic farms were
generally 20% lower. However, there was an offsetting decrease of 34% in fertilizer expense, a 53% reduction in energy costs, and a 97% decrease in pesticides. Reganold et al., (1987) reported a comparison of the long-term effects (40 years) of conventional farming to organic systems found that organic farms had significantly higher levels of soil organic matter than conventional systems, greater top soil depth, higher polysaccharide content, and less soil erosion. Clark et al., (1998) reported that over an eight year period of applying organic crop rotation practices that soil organic matter had increased 2% over a comparable field that used conventional practices in a two-year rotation scheme.
A major challenge to organic crop production is control of weeds. Weed management has
been identified by producers as the principle problem in organic farming (Walz, 1999). The advent and subsequent extensive use of herbicides after World War II altered crop
production practices, especially crop rotations. Conventional crop farming today usually involves two- or three-crop crop rotations as have been previously mentioned with a heavy reliance on herbicides to at least reduce or eliminate weed problems. Regardless of the type of farming system used, conventional or organic, weeds are a constant annual drag on
achieving maximum yields of high quality produce. Nave and Wax (1971) reported a
reduction in soybean seed yields of between 25% to 30% compared to weed free plots due to 38
Agricultural Science
the presence of one smooth pigweed ( Amaranthus hybridus L.) per 30 cm of row and that yield losses from stubble, lodging and stalks were more than double in pigweed and giant
foxtail ( Setaria fabric Herrm.) infested plots compared to weed free plots. Weeds compete with a crop for water, light, and soil nutrients directly reduce yields. Some species have alleopathnogenic effects upon certain crops, reducing their growth and yield or in extreme cases causing their death. Weeds can harbor insect pests or serve as alternate hosts to plant diseases that further reduce yields and produce quality. They can add off-flavors to crop products or in some cases provide toxins to produce, rendering it unhealthy to consume.
Weeds present during harvest can also damage the crop being harvested. Ellis et al., (1998) reported increases in damaged soybean seeds by 8.2% to 11.1% with the presences of a plant per m of row of any of five common weed species found in the Mid South. In organic
farming, crop rotations are vital to controlling weed growth. Teasdale et al., (2004)
evaluated the weed seed dynamics of three organic crop rotations and found that seedbanks of smooth pigweed and common lambsquarter ( Chenopodium album L.) were usually lower following a hay sward in a four-year rotation or wheat in a three-year rotation than
following soybean in a two-year rotation prior to being planted to corn. However, annual grassy weed seedbanks prior to corn planting were generally higher following the hay
sward of the four-year rotation than the wheat of three-year rotation or the soybean of the two-year rotation. Porter et al., (2003) also found that weed control in organic corn and soybean were better when they were part of a four-year rotation of corn-soybean-oat-alfalfa compared to a two-year corn-soybean scheme.
Nitrogen is probably the most important of the macro-nutrients in crop production.
Libraries at all major agricultural universities have a seemingly endless supply of research articles and texts pointing out the importance of adequate N-fertility in the growth and
development of every crop important to humankind. In organic crop farming, and
increasingly in conventional cropping systems, the use of crop rotations that supply ample supplies of N to non-leguminous cereals is an important management strategy. Prior to the escalating energy prices and the increasing cost of manufactured N-fertilizer sources, the use of legumes that fix atmospheric N by Rhizobium-legume symbiosis, especially clover species, as green manures or cover crops had diminished sharply. Even prior to World War I legumes would almost always be seeded for a green manure crop preceding corn (Heichel
and Barnes, 1984). In organic farming, where the emphasis is to refrain from using
manufactured N-fertilizer, green manure crops or cover crops are a vital source of N for
much of their production. Lupwayi et al., (1998) reported that microbial diversity was
greater under wheat preceded by red clover manure or field peas ( Pisum sativum L.) than under continuous wheat. Long-term crop rotation research in Iowa has shown very little or no increase in corn yields from plots receiving N-fertilizer compared to those following one or two years of an alfalfa- bromegrass- red clover meadow (Voss and Shrader, 1984).
Species selection for organic crop rotations is probably more thought out than for
conventional crop rotations. As explained earlier, conventional crop rotations are heavily influenced by market price of the various commodities available to be grown. Though
commodity price is important to practitioners of organic farming it is not the primary
driving force in making species selections. Baldwin (2006) states that organic farmers face the challenge of practicing crop rotations by defining systems that maintain farm profits while improving soil quality and preserving the environment. Frequently organic farmers
Concepts in Crop Rotations
39
will develop rotation schemes and select species based upon the crop’s ability to extract nutrients and water from the soil. Some organic producers include vegetable crops into
their operations due to these species tendency to extract nutrients and water from shallow depths then follow with a cereal that generally feeds to greater soil depths. Species selection is also made on the basis of weed, insect, and disease control. Delate and Hartzler (2003), states that rye has allopathic properties and is often used as a winter cover crop following corn to aid in weed control in preceding soybean or oat crops. Sustainability through the natural preservation of soil fertility is paramount to the organic farmer and makes the
selection of species an important part of the operation. Organic crop production makes as much use of natural pest control, including crop rotations, as is possible.
8. Biofuels production and crop rotations
Interest in using lignocellulosic biomass to produce ethanol is gaining in popularity.
Lignocellulosic biomass production mainly involves growing and harvesting plants
generally not used for food or feed. Woody species such as willow ( Salix. spp.) and poplars ( Populus spp.) (Matthew et al., 2010) and grasses such as switchgrass ( Panicum virgatum), big bluestem ( Andropogon gerardii), reed canarygrass ( Phalaris arundinacea) are several sources of lignocellulosic biomass that have shown to be useful in ethanol production (Hill, 2007).
Rotation schemes for growing lignocellulocsic crops are, for the most part, still in
development. Production of these materials for biofuels though is being done mostly on
land not suitable for extensive corn and soybean production thus relieving pressure to grow more hectares of these crops to satisfy the conventional and biofuels markets. Worldwide it is estimated that about 1% of crop land or about 11-12 million hectares are being used to grow biofuels (de Fraiture et al., 2008). Raghu et al., (2006) points out that some of the traits favorable to producing a lignocellulosic crop such as being a C4 photosynthetically, lacking pests, rapid early season growth, and long canopy duration can also tend towards them
being invasive. This would not work well in a rotation scheme with most conventional
crops. Currently lignocellulosic crops contribute little to current U.S. transportation biofuel suppliers but will likely provide the great share of ethanol in the near future.
In the United States, debate is underway concerning the use of corn as a primary source of fuel ethanol. Also, soybean oil is being blended with diesel to extend it. Diversion of these crops for biofuels is believed to increase food prices for consumers as the competing interest of food and fuel vie for the available supply. There is also concern that the increased
demands for these grains will negatively impact crop rotations, particularly those that help conserve soil, water, and plant nutrients. One of the primary reasons corn and soybean are currently popular for biofuels over lignocellulosic crops is the comparatively short time to harvest maturity. Most of the lignocellulosic crops require three to five years to reach harvest maturity(Hill, 2007), compared to one year for corn or soybean. Though corn and
soybean are regularly rotated with one another, history has shown that a substantial
increase in the price received for any crop can encourage monocultures at the expense of
proven rotations and their accompanying benefits. The expanded use of corn and soybean
as biofuel could diminish the inclusion of small grains and/or forage crops in rotation
schemes and the tillage of soils that are not well suited for cultivation.
Besides lignocellulosic crops, the harvesting of crop residues for ethanol production has been considered. Perlack et al., (2006) has reported that nearly 7.0 X 1010 kg of corn stover 40
Agricultural Science
could be harvested in the U.S. for ethanol production. Worldwide other crops that produce sufficient quantities of residue that could be used to produce ethanol include rice, barley, oat, wheat, sorghum, and sugar cane ( Saccharum officinarum L.). The use of crop residues for lignocellulosic ethanol production has however run into opposition due to the negative
impacts such removals have on C sequestration, soil properties, and nutrient availability for subsequent crops. Wilhelm et al., (2007) reported that between 5.25 and 12.50 Mg ha-1 of corn stover are required to maintain soil C at productive levels for subsequent crops. Lal (2004) states that even though the energy acquired from the world’s crop residue would be equivalent to 7.5 billion barrels of diesel, a 30% to 40% removal of crop residue would
increase soil erosion and its subsequent pollution hazards, deplete soil organic C, and
increase CO2 and other greenhouse gas emissions from the soil. He suggests establishing
biofuel plantations of adapted species on marginal lands rather than remove crop residues from land used to grow food and feed grains. Development of such plantations will require more aggressive research into developing crop rotation schemes specific for growing
lignocellulosic crops for biofuel.
9. Conservation tillage
Conservation tillage continues to grow in importance in crop production since its inception over 40 years ago. C.M. Woodruff in 1970 was conducting research on strip-planting corn
into tall fescue sod on Missouri hillsides with the idea of producing a cash grain/feed crop along with a forage crop for livestock production while maximizing conservation of the soil and rain water (Anonymous, 1970). Anders et al., (2004) found that phosphorus (P)
concentrations in run-off water were higher for no-till rice than conventional tilled paddies, most likely due to the P being surface applied. However, total-P concentrations in run-off were lower in no-till because of the reduced loss of soil in no-till and its bound P.
Conservation tillage which includes both minimum tillage and no-till practices, are used to grow an array of crops from corn, soybean, cotton, grain sorghum, and several small grain species almost always in some rotation scheme. It is popular not only for the conservation of natural resources as just mentioned, but also for the savings in fuel, time, wear and tear on farm equipment, and the environmentally sustainable attributes of the various practices.
Minimum tillage, by reduced soil disturbance, promotes a complex decomposition
subsystem that enhances soil system stability and efficiency of nutrient cycling. Basically minimum tillage more closely mimics natural ecosystems than conventional cropping
systems (Francis and Clegg, 1990). Tillage has been reported to reduce the diversity of
bacteria in the soil by reducing both the substrate richness and evenness (Lupwayi et al., 1998). They found that the influence of tillage on microbial diversity in fields planted to wheat was more prominent at the flag-leaf stage of growth than at seeding and more
prominent in bulk soil than in the rhizosphere at the flag-leaf stage.
Kladivko et al., (1986), studied the production of corn and soybean using an array of tillage systems ranging from conventional moldboard plowing and seedbed preparation to no-till
on a range of soils differing in organic matter, texture, and slope for seven-year and six-year periods. At one location on a Chalmers silty clay loam (fine-silty, mixed, superactive, mesic Typic Endoaquolls) comparisons of a corn-soybean rotation to continuous crops of these two species using various tillage systems was conducted for 10 years (Table 7). Yields of rotated crops tended to be greater than those of the monocultures regardless of tillage. Kladivko et Concepts in Crop Rotations
41
al. (1986) also reported from this research at other locations that conservation tillage systems resulted in increased soil water contents, lower soil temperatures, increased soil organic matter, and more water-stable aggregates near the soil surface with higher bulk densities than conventional tillage. Corn yields were found to be equal to or better than conventional tillage practices when grown on the better drained soils using conservation tillage. Only on the poorly drained soils did corn yields on conservation tilled fields fail to exceed
conventional tillage, most likely due to low temperatures and excess wetness in the spring as depicted with the Chalmers silty clay in Table 7.
Tillage
Previous
crop
Corn Soybean Soybean Corn
System
Crop
Corn
Corn
Soybean
Soybean
Fall plow
10.7
11.6
3.6
3.8
Fall chisel
10.3
11.4
3.3
3.6
Ridge till
10.4
11.6
3.4
3.6
No-till
9.1
11.2
3.2
3.3
Table 7. Mean corn and soybean yields (Mg ha-1) in response to tillage system and crop
rotation on a Chalmers silty clay loam in Indiana in 1980-1984 (data is from 6th to 10th year of the study). (Kladivko et al., 1986).
Roth (1996) presents several crop rotation schemes to use in no-till farming on Pennsylvania dairy farms. One of the more popular is an alfalfa-grass sward for hay followed by no-till corn. This involves killing the sod in the fall with herbicides to control weeds and reduce residue by early spring to facilitate corn planting. This rotation seems to work best where hay production is limited to three years. Alfalfa is also successfully no-tilled into fields that have just been harvested for corn silage or following the harvest of a spring seeded sorghum sudangrass. Lafond et al., (1992), evaluated no-till, minimum till (one pre-seeding tillage operation) and conventional till (fall and spring pre-seeding tillage operations) on a four-year crop rotation study. The rotations were fallow-spring wheat- spring wheat-winter
wheat, spring wheat-spring wheat-flax ( Linum usitartissimum L.)-winter wheat, and spring wheat-flax-winter wheat- field pea. Tillage systems did not affect the amount of water
conserved during fallow. However, no-till and minimum till did result in an increase in soil water from the surface to 120 cm in depth over conventional till. All three crops in the study had greater yields in the no-till and minimum till treatments than in the conventional till. In an experiment using conservation tillage practices (strip-till or no-till) in combination with a corn-soybean rotation, both full-season soybean or double-crop soybean following wheat
had the most consistent increase in seed yields (Edwards et al., 1987).
In recent years there has been considerable interest in various tillage practices and their influence on the sequestration of atmospheric CO2 as a partial means of mitigating its
current increase and subsequent impact on climate change. Sampson and Scholes (2000),
state that the optimization of crop management to facilitate accumulation of soil organic matter could help sequester atmospheric CO2 and lower the rate of its increase. West and
Post (2002), found that, excluding a change to no-till in wheat-fallow rotations, a change from conventional tillage to no-till can sequester between 43 to 71 g C m-2 yr-1. These
values are within the upper range (10 to 60 g C m2 yr-1) of those reported in a review by Follet (2001). West and Post (2002) also stated that enhanced crop rotation complexity can sequester an average of 8 to 32 g C m-2 yr-1 which is similar to an average of 20 g C m-2 yr-1
42
Agricultural Science
estimated by Lal et al. (1998; 1999) resulting from an improvement in rotation
management.
Conservation tillage can present pest control problems that are different from those found in conventional systems, particularly weeds. Weed species composition and abundance often
change in response to crop and soil management practices (Cardina et al., 2002). Buhler
(1995), wrote that most conservation tillage practices rely heavily on increased herbicide use and that reduced herbicide efficacy has slowed the adoption of conservation tillage
practices. Weed populations have tended to shift more towards perennials, summer annual
grasses, biennials and winter annual species in conservation tillage systems. Moyer et
al.,(1994) stated that successful conservation tillage systems usually involve crop rotations of three or more species and several different herbicides. Legere et al., 1997 concluded that conservation tillage has the potential to produce sustained yields of spring barley in Quebec, provided attention is given to critical aspects such as crop establishment and weed
management. With respect to plant diseases, Peters et al., (2003) determined that soil
agroecosystems can be modified by crop rotation and conservation tillage to increase
disease suppression by enhanced antibiosis abilities of endophytic and root zone bacteria in spring barley and potato ( Solanum tuberosum L.).
10. Conclusions
Crop rotation is very likely to continue to be an important management practice, especially in the developed part of the world. However, economics is always going to have a major
say in the decision making process of what gets planted where and by how much. Our ever
increasing world population is going to place greater pressure on getting more production from our shrinking areas of arable land and potable water needed for drinking, personal
use, and growing crops. Climate change is, despite all of the predictive computer models, a great unknown, not from the standpoint of whether or not it is occurring but as to just what can be realistically done, if anything, to curb it.
Lands that include a fallow period and/or irrigation to produce crops may eventually be
taken out of the food and fiber production equation because of both shifts in the climate and the loss of water for irrigation. Production areas of various crop species may shift due to climate change. Changes in crop genetics through biotech and genetic engineering
may contribute some relief but improvements in water use efficiency are not going to
eliminate the need for irrigation. Also, genetically engineered crops in many areas of the world are not being well received based on fears, real or imaginary, and increased costs of such seed stocks are often prohibitive to many producers. A nearly 500% increase in
energy prices and a shift from food, feed, and fiber crops to renewable energy crops will have an increasing impact on the land available to grow all crops and the rotations to
produce them. Given the history of crop rotations and the overall benefits that appear to be gained from them, it is virtually assured that they will continue to be an important
practice in food production. Despite the difficulties associated with conducting crop
rotation research, it will be beneficial to society as a whole to support efforts in this area and to adequately reward scientists willing to dedicate their professional efforts in such endeavors.
Concepts in Crop Rotations
43
11. References
Anders, M., Olk, M.D., Harper, T., Daniel, T, & Holzauer, J. (2004). The effect of rotation, tillage, and fertility on rice grain yields and nutrient flows. Proceedings 26th
Southern Conservation Tillage Conference for Sustainable Agriculture. 8-9 June. North Carolina State University, Raleigh, NC. pp 26-33 .
Anony