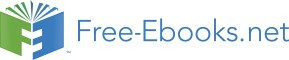

INTRODUCTION
Plants have evolved a number of inducible defense mechanisms against pathogen attack. Recognition of a pathogen often triggers a localized resistance reaction, known as the hypersensitive response (HR), which is characterized by rapid cell death at the site of infection. In the 1960s, research showed that tobacco plants challenged with tobacco mosaic virus (TMV) subsequently developed increased resistance to secondary infection in distal tissues. This spread of resistance throughout the plant's tissues was termed systemic acquired resistance (SAR). We now know that SAR can be activated in many plant species by pathogens that cause necrosis, either as part of the HR or as a symptom of disease. The resistance conferred is long-lasting, sometimes for the lifetime of the plant, and effective against a broad-spectrum of pathogens including viruses, bacteria, fungi, and oomycetes. More recently, within the last 5 years or so, research has shown that it is possible for turf grass plants to achieve systemic acquired resistance.
Molecularly, SAR is characterized by the increased expression of a large number of pathogenesis-related genes (PR genes), in both local and systemic tissues. PR proteins were first described in the 1970s by Van Loon, who observed accumulation of various novel proteins after infection of tobacco with TMV. Although many PR proteins have antimicrobial properties in vitro, the function of each in the defense response has not been clearly defined. It is generally thought that SAR results from the concerted effects of many PR proteins rather than a specific PR protein. Although their roles in establishing SAR are unclear, PR genes serve as useful molecular markers for the onset of SAR.
In 1979, White observed that PR protein accumulation and resistance to TMV could be induced by treatment of tobacco with salicylic acid (SA), aspirin (acetyl SA), or benzoic acid. Evidence that SA is a signal for the induction of SAR came from two studies published in 1990. Studies showed that the endogenous SA concentration rises in both local and systemic tissues after infection of tobacco with TMV and this rise correlates with PR gene inductiction. Researchers found that cucumber plants infected with either Colletotrichum lagenarium or tobacco necrosis virus (TNV) have considerably elevated levels of SA in the phloem sap. In a search for SA analogues that were less phytotoxic than SA, 2,6-dichloroisonicotinic acid (INA) and benzothiadiazole S-methyl ester (BTH) were found to induce the same set of PR genes. A requirement for SA as an endogenous signal for SAR was proven by Gaffney et al. using a bacterial gene, nahG, encoding salicylate hydroxylase, which removes SA by conversion to catechol. Transgenic tobacco and Arabidopsis expressing nahG accumulate very little SA after pathogen infection, fail to express PRgenes, and are impaired in SAR (17, 35).
In the past 10 years, genetic analyses in the model plant Arabidopsis have identified additional components of SAR downstream of SA. Plants that are nonresponsive to SA were identified in a number of mutant screens and found to have mutations in the same gene, NPR1/NIM1 (NON-EXPRESSER OF PR GENES1/NONINDUCIBLE IMMUNITY1). Considerable progress has been made in elucidating the role of NPR1 and associated proteins in the induction of SAR since the last Annual Review on SAR in 1997. We therefore focus on these recent molecular and genetic experiments that have contributed to our understanding of SAR.