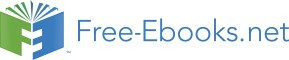

CASE
1
Sweep and Swing: Reshaping the Wing for the Jet and Rocket Age
The development of the swept and delta wing planform enabled practical attainment of the high speeds promised by the invention of the turbojet engine and the solid-and-liquid-fueled rocket. Refining the swept and delta planforms from theoretical constructs to practical realities involved many challenges and problems requiring creative analysis and study by NACA and NASA researchers. Their insight and perseverance led to the swept wing becoming the iconic symbol of the jet age.
Case-1 Cover Image: The X-48B subscale demonstrator for the Blended Wing-Body (BWB). The BWB may represent the next extension of the swept and delta wing, to transform flight away from the rule of the “tube and wing” jetliner. NASA.
The progressive evolution of aircraft design has witnessed continuous configuration changes, adaptations, and reinterpretations. The canard wood-and-fabric biplane launched the powered flight revolution and gave way to the tractor biplane and monoplane, and both gave way to the all-metal monoplane of the interwar era. The turbojet engine set aside the piston engine as the primary motive power for long-range commercial and military aircraft, and it has been continually refined to generate the sophisticated bypass turbofans of the present era, some with afterburning as well. The increasing airspeed of aircraft drove its own transformation of configuration, measurable in the changed relationship between aspect and fineness ratios. Across the primacy of the propeller-driven era, from the beginning of the 20th century to the end of the interwar era, wingspan generally far exceeded fuselage length. That changed early in the jet and rocket era. By the time military and test pilots from the National Advisory Committee for Aeronautics (NACA) first probed the speed of sound with the Bell XS-1 and Douglas D-558-1 Skystreak, wingspan and fuselage length were roughly equal. Within a decade, as aircraft speed extended into the supersonic regime, the ratio of wingspan to fuselage length dramatically reversed, evidenced by aircraft such as the Douglas X-3, the Lockheed F-104 Starfighter, and the Anglo-French Concorde Supersonic Transport (SST). Nicknames handily captured the transformation: the rakish X-3 was known informally as the “Stiletto” and the only slightly less sleek F-104 as the “Missile with a Man in It.”
There was as well another manifestation of profound design transformation, one that gave to the airplane a new identity that swiftly became a global icon: the advent of the swept wing. If the biplane constituted the normative airplane of the first quarter century of flight and the straight wing cantilever monoplane that of the next quarter century, by the time of the golden anniversary of Kitty Hawk, the swept wing airplane had supplanted both, its futuristic predominance embodied by the elegant North American F-86 Sabre that did battle in “MiG Alley,” high over North Korea’s blue-gray hills bordering the Yalu River. In the post-Korean era, as swept wing Boeing 707 and Douglas DC-8 jet airliners replaced what historian Peter Brooks termed the “DC-4 generation” of straight wing propeller-driven transports, the swept wing became the iconic embodiment of the entire jet age.[1] Today, 75 years since its enunciation at an international conference, the high-speed swept wing is the commonly accepted global highway symbol for airports, whether an intercontinental center such as Los Angeles, Frankfurt, or Heathrow; regional hubs such as Dallas, Copenhagen, or Charlotte; or any of the myriad general aviation and business aviation airfields around the world, even those still primarily populated, ironically, by small, straight wing propeller-and-piston-driven airplanes.
The Tailless Imperative: The Early History of Swept and Delta Wings
The high-speed swept wing first appeared in the mid-1930s and, like most elements in aircraft design, was European by birth. But this did not mark the swept wing’s first appearance in the world’s skies. The swept wing dated to before the First World War, when John Dunne had developed a series of tailless flying wing biplanes using the swept planform as a means of ensuring inherent longitudinal stability, imparting “self-correcting” restoration of any gust-induced pitching motions. Dunne’s aircraft, while freakish, did enjoy some commercial success. He sold manufacturing rights to the Burgess Company in the United States, which subsequently produced two “Burgess-Dunne” seaplanes for the U.S. Navy. Lt. Holden C. Richardson, subsequently one of the first members of the NACA, had urged their purchase “so that the[ir] advantages and limitations can be thoroughly determined . . . as it appears to be only the beginning of an important development in aeronautical design.”[2]
That it was, though not in the fashion Richardson expected. The swept wing remained an international staple of tailless self-stabilizing design, typified in the interwar years by the various Westland Pterodactyl aircraft designed by Britain’s G.T.R. Hill, the tailless aircraft of Boris Ivanovich Cheranovskiy, Waldo Waterman’s Arrowplane, and a series of increasingly sophisticated sailplanes and powered aircraft designed by Germany’s Alexander Lippisch. However, it would not become the “mainstream” element of aircraft design its proponents hoped until applied to a very different purpose: reducing transonic aerodynamic effects.[3] The transonic swept wing effectively increased a wing’s critical Mach number (the “drag divergence Mach number”), delaying the onset of transonic drag rise and enabling an airplane to fly at higher transonic and supersonic speeds for the same energy expenditure and drag penalty that a straight wing airplane would expend and experience at much lower subsonic speeds.
In 1935, leading aerodynamicists gathered in Rome for the Volta Congress on High Speeds in Aviation, held to coincide with the opening of Italy’s impressive new Guidonia laboratory complex. There, a young German fluid dynamicist, Adolf Busemann, unveiled the concept of using the swept wing as a means of attaining supersonic flight.[4] In his presentation, he demonstrated the circulation pattern around a swept wing that, essentially, “fooled” it into “believing” it was flying at lower velocities. As well, he presented a sketch of an aircraft with such a “Pfielförmiges Tragwerk” (“Arrow-Shaped Lifting Surface”), though one that had, by the standards of subsequent design, very modest sweep and very high aspect ratio.[5]
Theodore von Kármán recalled not quite two decades later that afterward, at the conference banquet, “General [Arturo] Crocco, the organizer of the congress and a man of far-reaching vision, went further while doodling on the back of the menu card, drawing a plane with sweptback wings and tail, and even swept propeller blades, laughingly calling it ‘Busemann’s airplane.’”[6] Evidence exists that Crocco took the concept beyond mere dinner conversation, for afterward, Guidonia researchers evaluated a design blending modestly swept wings with a “push-pull” twin-engine fuselage configuration. However, Guidonia soon returned to the more conventional, reflecting the Italian air ministry’s increasing emphasis upon building a large and powerful air arm incorporating already proven and dependable technology.[7]
Delegates from other nations present at Busemann’s briefing missed its significance altogether, perhaps because his gently swept configuration—in the era of the DC-2 and DC-3, which had pronounced leading edge taper—looked far less radical than the theory and purpose behind it implied. NACA Langley Memorial Aeronautical Laboratory researchers had already evaluated far more sharply swept planforms at Langley for a seminal wing taper study the laboratory issued the next year.[8] Thus, at first glance, Busemann’s design certainly did not look like a shape that would transform aviation from the firmly subsonic to the transonic, making possible the potential of the jet engine, and the jet age (with its jet set) that followed.
Therefore, for the United States and most other nations, over the next decade, the normative airplane remained one having straight (if tapered) wings and piston propulsion. For Germany, however, the future belonged to increasingly sharply swept and delta wings, and jet and rocket propulsion as well. Within 5 years of the Volta conference, with Europe engulfed in a new war, its engineers had already flown their first jet and rocket-powered aircraft, had expanded beyond Busemann’s initial conception to derive shapes more closely anticipating subsequent high-speed aircraft and missile designs, and were busily testing models of swept wing transonic airplanes and supersonic missiles. Lippisch’s swept wing sailplanes had presaged a new Messerschmitt rocket-propelled interceptor, the Me 163 Komet (“Comet”), and his broad, high aspect ratio deltas had given way to a rounded triangular planform that he envisioned as meeting the needs for transonic and supersonic flight. While many of these concepts by Lippisch and other German designers were impracticable, or unrelated to Germany’s more immediate military needs, others possessed significant military or research potential. Only flawed decisions by the Third Reich’s own leadership and the Allies’ overrunning of Germany would prevent them from being developed and employed before the collapse of the Hitler regime in May 1945.[9]
Birthing the American Delta and Swept Wing
The extent to which the swept wing permeated German aeronautical thought understandably engendered tremendous postwar interest in the benefits of swept planforms for transonic and supersonic flight within the American, European, and Soviet aeronautical communities.[10] However, for America, uncovering German swept wing research and development furnished the confirmation of its value, not its discovery, for Robert T. Jones, an aerodynamicist at the Langley Memorial Aeronautical Laboratory, had independently discovered its benefits in 1944, a year before the Allies first entered Germany’s shattered and shuttered research laboratories and design shops.[11]
A sampling of various design concepts for Lippisch swept wing and delta aircraft. These original Lippisch sketches were incorporated in “German Aircraft: New and Projected Types,” a 1946 Allied technical intelligence summary. USAF.
The Gluhareff-Griswold Nexus
In 1936, Michael E. Gluhareff, an emigree Russian engineer who was chief of design for the Vought-Sikorsky Aircraft Division of United Aircraft Corporation, began examining various tailless aircraft configurations. By July 1941, his study had spawned a proposed interceptor fighter powered by a piston engine driving a contra-rotating pusher propeller. It had a rounded delta planform resembling an arrowhead, with leading edges swept aft at 56 degrees. It featured a tricycle retractable landing gear, twin ventral vertical fins, an extremely streamlined and rounded configuration, provisions for six heavy machine guns, and elevons (combined ailerons and elevators) for roll and pitch control. Gluhareff informed company founder Igor I. Sikorsky that its sharp sweep would delay the onset of transonic compressibility, noting, “The general shape and form of the aircraft is, therefore, outstandingly adaptable for extremely high speeds.”[12]
In retrospect, Gluhareff’s design was a remarkable achievement, conceived at just the right time to have been completed with turbojet propulsion (for which its configuration and internal layout was eminently suited) though circumstances conspired against its development. Sikorsky was then perfecting the first practical helicopter—the VS-300, another revolutionary development, of course—and chose understandably to concentrate on rotary wing flight. He did authorize Gluhareff to solicit support from inventor-entrepreneur Roger W. Griswold, president of the Ludington-Griswold Company, about building a wind tunnel model of the configuration.[13] Tests by United Aircraft proved so encouraging that Griswold approached the engineering staff of the Army Air Forces (AAF) at its Wright Field Aircraft Laboratory about sponsoring what was now called the “Dart.”[14] But having their fill of visitors bringing a series of the weird and unconventional, and charged with ensuring that the AAF acquired large numbers of aircraft, and quickly, the AAF’s engineers did not pursue the project.[15]
The proposed Gluhareff Dart fighter of 1941, showing both its novel layout and, for the time, nearly as novel tricycle landing gear layout. National Air and Space Museum, Smithsonian Institution.
So the Gluhareff-Griswold Dart never reached the hardware stage, the failure to build it counting as a loss to American midcentury aeronautics. As for Gluhareff, though he had made notable contributions to Sikorsky’s large flying boats (and would, as well, to his helicopters), he continued to explore the basic design of his intriguing if abortive configuration, proposing a variety of derivatives, including in 1959 a Mach 2+ supersonic transport with a small canard wing and double-deck fuselage.[16] If the Dart never saw development, its configuration nevertheless proved significant. In 1944, Griswold resurrected the Dart shape for a proposed 2,000-pound guided glide bomb, or “glomb.” The Army Air Forces recommended he obtain the NACA’s opinion of its aerodynamics, and for this, Griswold turned to Langley Memorial Aeronautical Laboratory. There, on August 19, he met with the NACA’s resident aerodynamic expert on “pilotless missiles,” Robert T. Jones. Out of that contact would emerge both the American delta and swept wing.
Enter Robert T. Jones . . .
“R.T.” Jones was a brilliant, flight-obsessed, and largely self-taught fluid dynamicist, having dropped out of the University of Missouri to join a flying circus, then working as a designer for Nicholas-Beazley, a small Missouri aircraft company. When the Great Depression collapsed the firm, his father used political connections as Chairman of the local Democratic Party to secure Jones a job running elevators in the U.S. Capitol. In his spare time and evenings, he studied mathematics and aerodynamics with Albert Zahm, the aeronautics Chair at the Library of Congress, and with Max Munk at Catholic University. Despite his lack of a formal engineering degree, through the efforts of Representative David Lewis (a homespun Maryland progressive with a strong interest in self-improvement who had taken math instruction from the young elevator operator), Jones received a temporary appointment as a “scientific aide” to the NACA. There, he quickly proved such a gifted and insightful researcher that he soon secured a coveted permanent position at Langley, consorting with the likes of John Stack, Eastman Jacobs, and Theodore Theodorsen.[17]
As he considered Griswold’s “glomb,” Jones recognized that its extremely low aspect ratio shape (that is, a shape having a very long wing root in relation to its total wingspan) could not be adequately analyzed using conventional Prandtl-rooted “lifting line” theory. Instead, Jones drew on the work of his mentor Munk, using papers that Munk had written on the flow of air around inclined airship hulls and swept wings, and one by the Guggenheim Aeronautical Laboratory’s Hsue-shen Tsien, a von Kármán associate at the California Institute of Technology (Caltech), on airflow around inclined bodies of revolution. He analyzed it using linear equations governing two-dimensional incompressible flow, considering his results of little practical value, recalling three decades later, “I thought, well, this is so crude, nobody would be interested. So I just hid it in my desk.”[18]
But it sparked his curiosity, and in January 1945, by which time he was busy thinking about nonlinear compressible flows, he had a revelation: the equations he had developed months earlier for the glomb analysis could be applied to a low aspect triangular wing operating in supersonic flow, one whose wing-leading edges were so sharply swept as to place them within the shock cone formed around the vehicle and hence operating in subsonic flow. In these conditions, the wing was essentially “fooled” into behaving as if it were operating at a much lower Mach number. As Jones recalled, “It finally dawned on me that the slender wing theory would hold for compressible flow and even at supersonic speed if it were near the center of the Mach cone. So, I immediately got the paper out and I added the compressible flow parts to it, which was really the important part, and then I wondered well, why is it that this slender wing doesn’t have an effect on compressibility? Then I realized that it was because the obliquity of the edge and that this is the simple sweep theory and would work in spite of the compressibility effect. So, I wrote a paper which incorporated the slender wing theory and also sweep theory.”[19] Jones then moved from considering a slender triangular delta [Δ] to the sharply sweptback wing [^], the reverse of Germany, where the high-speed swept wing had preceded, not followed, the delta.[20]
Jones’s delta and swept wing utilized, for their time, very thin airfoil sections, ones typical of supersonic aircraft to come. In contrast, German swept and delta wing developer Alexander Lippisch had employed much thicker sections that proved unsuitable for transonic flight. His tailless rocket-propelled swept wing Me 163 Komet (“Comet”) interceptor, for example, essentially became uncontrollable at speeds slightly above Mach 0.85 thanks to stability changes induced by shock wave formation on its relatively thick wing. His design for a rocket-boosted, ramjet-powered delta fighter, the P 13, had such thick wing and tail sections—the pilot actually sat within the leading edge of the vertical fin—that it could never have achieved its desired transonic performance. As discussed subsequently, postwar NACA tests of a captured glider configuration of this design, the DFS DM-1, confirmed that transonic delta wings should be far thinner, with sharper leading edges. As a consequence, NACA researchers rejected the Lippisch approach, and, though some of them tried extrapolations of his designs (but with lower thickness-chord ratios and sharper leading edges), the NACA (and industry as well) adapted instead the thin slender delta, à la Jones.[21]
Dissemination, Deliberation, and Confirmation
In February 1945, Jones showed his notes on sweep to Jean Roché, the Army Air Forces technical liaison at Langley, and informed others as well, including Maj. Ezra Kotcher of the AAF’s Air Technical Service Command, and NACA colleagues Arthur Kantrowitz and Hartley A. Soulé.[22] Kotcher passed it along to von Kármán and Tsien—then working as scientific advisers to Gen. Henry H. “Hap” Arnold, the Army Air Forces’ Chief of Staff—and Soulé and Kantrowitz urged Jones to inform the Agency’s Director of Research, George W. Lewis, of his discovery.[23] Accordingly, on March 5, 1945, Jones informed Lewis, “I have recently made a theoretical analysis which indicates that a V-shaped wing travelling point foremost would be less affected by compressibility than other planforms. In fact, if the angle of the V is kept small relative to the Mach angle [the angle of the shockwave], the lift and center of pressure remain the same at speeds both above and below the speed of sound.”[24] Jones subsequently undertook tests in the Langley 9-inch supersonic tunnel of a small, 4-inch-long daggerlike sheet-steel triangular wing with rounded leading edges and a span of only 1.5 inches, tests that complemented other trials at Aberdeen, MD, arranged by von Kármán and Tsien.
The Langley tests, through the transonic region and up to Mach 1.75, confirmed his expectations, and Jones published his first test results May 11, 1945, noting, “The lift distribution of a pointed airfoil travelling point-foremost is relatively unaffected by the compressibility of the air below or above the speed of sound.”[25] This was almost 2 weeks before Lippisch informed von Kármán, then leading an AAF European study team, of his high-speed delta concepts (during a technical intelligence interrogation at St. Germain, France, on May 23), not quite a month before von Kármán assistant Clark Millikan visited the Messerschmitt advanced projects group at Oberammergau on June 9–10 and interrogated Waldemar Voigt about his swept wing fighter concepts, and well over a month before Millikan journeyed to Völkenrode to interrogate German swept wing inventor Adolf Busemann, on June 20–21.[26]
Langley’s peer reviewers and senior Agency official Theodore Theodorsen did not immediately accept Jones’s assumption that a unified slender wing theory could apply to both compressible and incompressible flows and even questioned the evidence of sweep’s benefits. Fortunately, Jones was greatly assisted in confounding skeptics by the timely results of NACA tunnel tests and falling body experiments, which left little doubt that sweep worked. As well, an associate of Jones made a most helpful discovery: locating a 1942 British translation of Busemann’s 1935 paper. Evidence of an enemy’s interest coincident with one’s own work always heightens its perceived value, and undoubtedly, the Busemann paper, however dated, now strongly bolstered Jones’s case. When it became time to assemble a bibliography for his swept wing report, Jones added Busemann’s paper and other German sources by Albert Betz, H.G. Küssner, Ludwig Prandtl, and Hermann Schlichting, though it is unclear whether this reflected a collegial respect across the chasm of war or simply a shrewd appreciation of their persuasive value.[27]
Langley released his report in late June 1945.[28] In it, Jones noted: “the attachment of plane waves to the airfoil at near-sonic or supersonic speeds (Ackeret theory) may be avoided and the pressure drag may be reduced by the use of planforms in which the angle of sweepback is greater than the Mach angle. The analysis indicates that for aerodynamic efficiency, wings designed for flight at supersonic speeds should be swept back at an angle greater than the Mach angle and the angle of sweepback should be such that the component of velocity normal to the leading edge is less than the critical speed of the airfoil sections. This principle may also be applied to wings designed for subsonic speeds near the speed of sound, for which the induced velocities resulting from the thickness might otherwise be sufficiently great to cause shock waves.”[29] Such marked the effective birth of the high-speed swept wing airplane in the United States, as his report weeks earlier had marked the birth of the American high-speed delta.
Jones showed these notes on the concept of high-speed wing sweep to Langley’s AAF technical liaison representative Jean Roché on February 27, 1945. NASA.
By the time Jones’s report appeared, Germany’s aeronautical establishment was already under the microscope of Allied technical intelligence, whose teams swiftly focused on its intensive investment in swept wing aerodynamics for its missiles and aircraft. Replicating reaction to the earlier “discovery” of “Göttingen aerodynamics” after the First World War, the post–Second World War influence of German example and practice was even more profound. Indeed, it affected the entire postwar course of European, Soviet, and American high-speed aerodynamic research, development, test, evaluation, and acquisition. In the increasingly tense national security environment of the burgeoning Cold War, the national intelligence services of the various advanced aeronautical nations understandably maintained very active technical collection efforts to learn what they did not already know.[30]
Swept Wing Challenges
The NACA so rapidly focused its attention on swept planforms that, within 2 years of the end of the Second World War, George Gray, author of a popular yet surprisingly detailed study of the Agency, could already write: “Just how far the sweepback principle can be applied with resulting advantage is a question. . . . At about 90 percent of the speed of sound both sweepback and low aspect ratio begin to be of value, and wings that combine the two features seem to offer a promising choice. At about Mach number 1.50, a sweepback of 60 degrees seems necessary to escape the backward flare of the Mach angle. . . . At Mach number 2.00, the angle is so acute that it is impossible to avoid it and still preserve the wings. It may be that designers preparing for flight at this speed will return to wings of low angles of sweep, and place their main dependence for drag reduction on thinning the profiles, lowering the aspect ratio, and sharpening the edges of wings.”[31] By 1950, this growing confidence in the old-new swept planform had resulted in transonic and supersonic research airplanes, a variety of military prototypes, and two operational jet fighters that would shortly clash over North Korea: the American F-86 Sabre (first flight in October 1947) and, in the Soviet Union, the MiG-15 (first flight in December 1947).[32]
The North American XP-86, prototype of the F-86 Sabre family, represented an amalgam of German and American swept wing and streamlined aerodynamics. USAF.
Swept wing aircraft, for all their high-speed advantages, posed daunting stability, control, and handling qualities challenges. Foremost of these was pitch-up at low and high speeds, resulting from deteriorating longitudinal stability.[33] A swept wing airplane’s lateral-directional stability was compromised as well by so-called “dihedral effects.” Swept wing aircraft with excessive dihedral experienced pronounced combined rolling and yawing “Dutch roll” motions, which would be unacceptable on both production civil and military designs.[34] Such motions would induce airsickness in passengers on large aircraft and, on bomber, fighter, and attack aircraft, prevent accurate tracking of a maneuvering target or accurate bomb release. (Indeed, it was largely because of this kind of behavior that the U.S. Air Force did not proceed with production of Northrop’s YB-49 flying wing jet bomber.) Adverse yaw posed another problem. At higher speeds, as a swept wing plane rolled from aileron deflection, it experienced higher drag and loss of lift involving the lowered wing, generating a tendency of the airplane to turn (reverse) into the direction of the raised wing, effectively doing the opposite of what the pilot intended. Adverse yaw could be caused by aeroelastic effects as well. That swept wing aircraft would possess behavior characteristics significantly different than conventional straight wing designs did not come as a surprise to the NACA or other aerodynamic researchers in America and overseas. But all recognized the need to complement theory and ground-test methodologies with flight research.
The peculiarities of swept wing aircraft, at a time when early jet aircraft lacked the power-to-weight advantages of later designs, could—and often did—prove fatal. For example, Boeing designed the B-47, America’s first large swept wing aircraft, with pod-mounted engines and a broad, highly tapered, thin swept wing. During flight-testing at higher speeds, test pilots found aileron input to roll the aircraft would twist the wing, the aileron effectively acting as a trim-tab does on a control surface. The twisted wing would overcome the rolling